Quantum Leap
In an exciting development for the field of quantum computing, researchers at Oxford University have made a groundbreaking advancement by successfully linking separate quantum processors through a photonic network interface. This breakthrough in distributed quantum computing represents a significant step toward overcoming the scalability problem that has long hindered the potential of quantum technology. By using photonic links to entangle qubits across different modules, the team has effectively created a fully connected quantum computer capable of performing complex computations. This innovative approach not only paves the way for the realisation of a quantum internet but also demonstrates the potential for high-performance quantum computers to tackle tasks that even the most advanced supercomputers struggle with today. Dive into this fascinating development to explore how Oxford’s achievement could redefine the future of computing.
Oxford’s Quantum Breakthrough
Oxford University’s recent achievement in quantum computing marks a significant milestone in the field. This section explores the core aspects of their breakthrough and its potential implications for the future of computing and communication.
Distributed Quantum Computing Unveiled
Oxford scientists have successfully demonstrated distributed quantum computing, a groundbreaking achievement that could revolutionize the field. This approach involves linking separate quantum processors through a photonic network interface, creating a fully connected quantum computer.
The team’s success lies in their ability to perform quantum computations across multiple modules, effectively overcoming previous limitations of single-device quantum computers. This distributed approach allows for greater flexibility and scalability in quantum systems.
By leveraging photonic links, the researchers have opened up new possibilities for quantum information processing, paving the way for more powerful and practical quantum computers in the future.
Overcoming the Scalability Problem
The scalability problem has long been a significant hurdle in quantum computing. Traditional approaches face limitations when trying to increase the number of qubits in a single device.
Oxford’s breakthrough addresses this challenge by using a modular approach. Instead of cramming millions of qubits into one massive machine, their method allows for connecting smaller quantum devices, each containing a manageable number of qubits.
This innovative strategy offers several advantages:
- Flexibility in system design
- Easier maintenance and upgrades
- Potential for unlimited scalability
- Reduced engineering complexities
By tackling the scalability issue, Oxford’s research brings us closer to realizing practical, large-scale quantum computers capable of solving complex problems beyond the reach of classical supercomputers.
Building a Quantum Internet
The Oxford team’s work lays the foundation for a future “quantum internet,” a network where distant quantum processors can communicate and perform computations securely.
This quantum internet could revolutionize various fields:
- Cryptography: Ultra-secure communication channels
- Distributed computing: Enhanced computational power across networks
- Sensing: Improved precision in measurements and detection
The potential for a quantum internet has sparked excitement in the scientific community, with researchers envisioning a new era of information processing and secure communication.
As development continues, we may see the emergence of a global quantum network, fundamentally changing how we approach computation, communication, and data security.
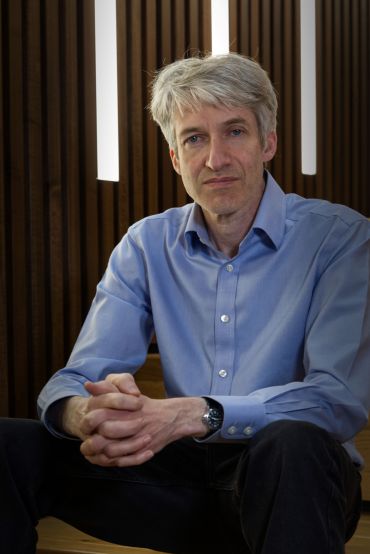
Core Components and Methods
Understanding the technical aspects of Oxford’s breakthrough is crucial to appreciating its significance. This section delves into the key components and methods that made this achievement possible.
Role of Qubits and Photonic Links
Qubits, the fundamental units of quantum information, play a central role in Oxford’s distributed quantum computing system. Unlike classical bits, qubits can exist in multiple states simultaneously, thanks to the principle of superposition.
The researchers used trapped-ion qubits, which are atomic-scale particles that can be manipulated to store and process quantum information. These qubits are housed in separate modules, each containing a small number of them.
The key innovation lies in the use of photonic links to connect these modules. These optical fiber connections use light (photons) to transmit data between modules, enabling entanglement and quantum operations across the network.
Quantum Teleportation: A New Milestone
Quantum teleportation is a crucial technique in the Oxford team’s breakthrough. It allows for the transfer of quantum information between distant qubits without physical movement of the particles themselves.
The researchers achieved a significant milestone by demonstrating quantum teleportation of logical gates across a network link. This means they could perform quantum operations between qubits in separate modules, effectively creating a single, cohesive quantum computer.
Key aspects of this achievement include:
- Entanglement of qubits across different modules
- Teleportation of quantum states and operations
- Preservation of quantum coherence during information transfer
This advancement in quantum teleportation opens up new possibilities for distributed quantum computing and the development of quantum networks.
Grover’s Search Algorithm in Action
To demonstrate the effectiveness of their distributed quantum computing system, the Oxford team implemented Grover’s search algorithm. This quantum algorithm is known for its ability to search unstructured databases much faster than classical algorithms.
Grover’s algorithm showcases the power of quantum computing in the following ways:
- It can find a specific item in a large dataset with fewer operations than classical methods.
- It leverages quantum superposition to explore multiple possibilities simultaneously.
- It demonstrates a quadratic speedup over classical search algorithms.
By successfully running Grover’s algorithm on their distributed system, the researchers proved that their approach could handle complex quantum computations across multiple modules. This achievement underscores the potential of distributed quantum computing to tackle problems that are intractable for classical computers.